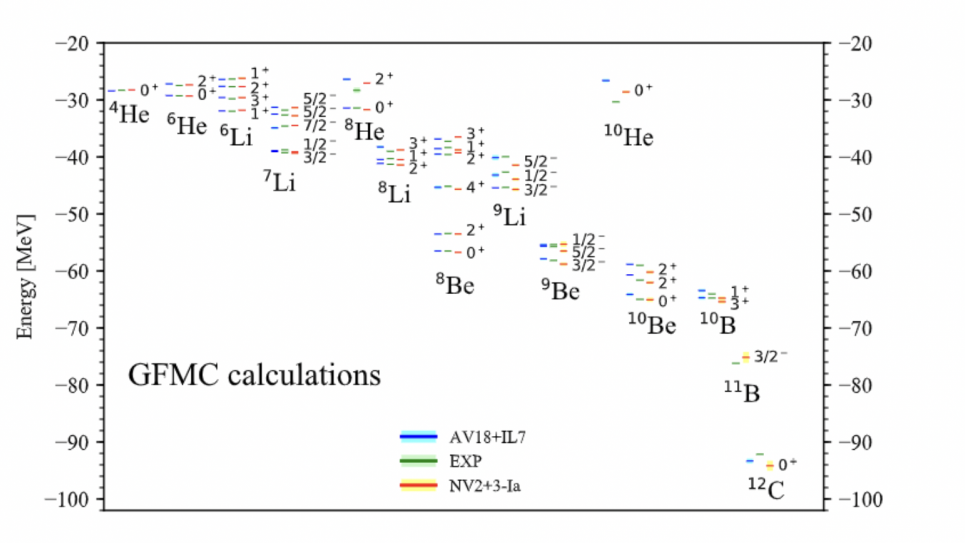
Energy spectra ofA= 4–12 nuclei obtained with the NV2+3-Ia chi-ral interactions compared to experimental data [45]. Also shown are results obtained with thephenomenological AV18+IL7 Hamiltonian.
The overall goal of this research is to accurately describe atomic nuclei (including their spectra, densities, structure functions, transitions, low-energy scattering, and responses) while simultaneously predicting the equation of state of neutron matter.
Nuclear physics stands at the core of several exciting developments in science: state-of-the-art experimental facilities are probing the structure and reactions of stable and radioactive nuclei; the emerging gravitational-wave astronomy has opened a new window on the state of matter under extreme conditions; atomic nuclei are used in current and planned neutrino-oscillation and double-beta decay experiments, which will measure neutrino properties with unprecedented accuracy. The above efforts are accompanied by a remarkable progress of microscopic nuclear approaches that describe nuclei and nucleonic matter starting from the individual interactions among protons and neutrons. These nuclear forces are rooted in quantum chromodynamics, the fundamental theory of strong interactions, and are the main input of numerical methods that use high-performance computers to solve the nuclear Schrodinger equation with controlled approximation.
To achieve the set objective, numerical quantum Monte Carlo methods will be extended to treat the 16O nucleus, which has proven to be most effective in constraining three-nucleon potentials, relevant for the stability of neutron stars. In addition, novel computational methods will be used to study nuclear response densities induced by electrons and neutrinos scattering and gain more exclusive information about these processes. These studies will impact experimental programs on gravitational waves carried out by, e.g., the LIGO-Virgo collaboration, ongoing experiments that use electrons to study in medium nucleon-correlations carried out at Jefferson Lab, and neutrino experimental facilities, e.g., the Deep Underground Neutrino Experiment (DUNE).