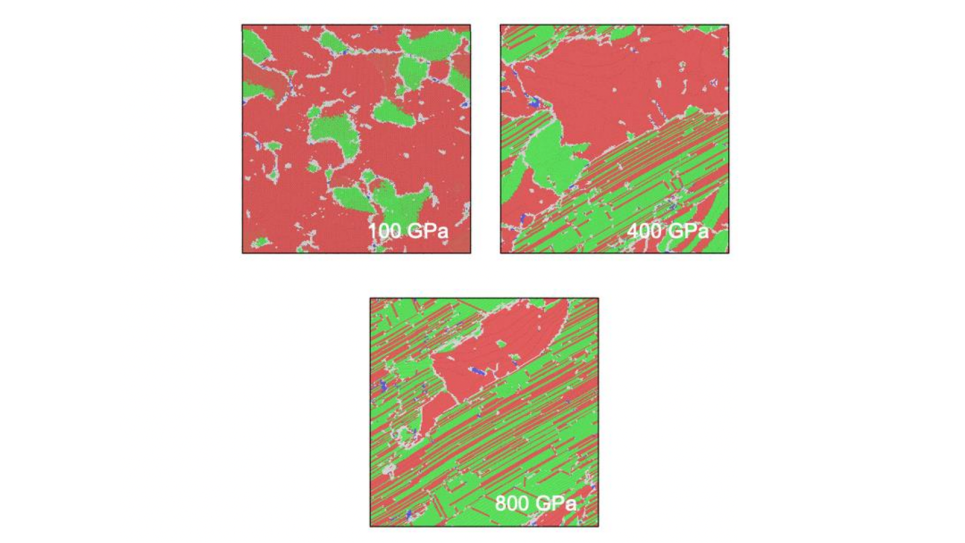
Results from preliminary MD <011> shock-ramp simulation using an inexpensive potential. The images are transverse sections of the simulation analyzed with polyhedral template matching to show the grain structure and phases. Red indicates hcp lattice; green, fcc.