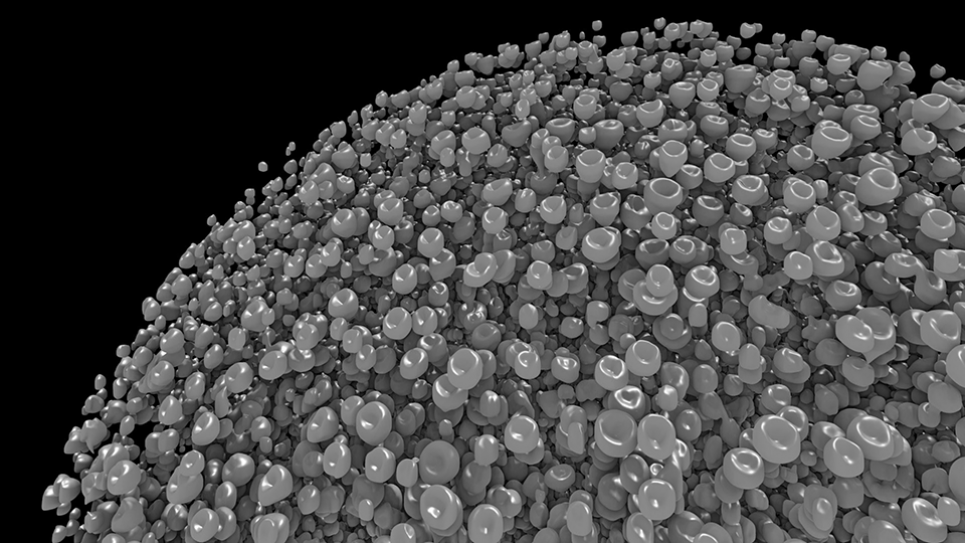
Cloud cavitation collapse—the evolution of clusters of vapor bubbles in high-pressure flow—is detrimental to the lifetime of high-pressure injection engines, yet beneficial to kidney lithotripsy and ultrasonic drug delivery. Despite its importance, researchers have limited understanding of the governing mechanisms necessary to design informed strategies for controlling it.
Cloud cavitation collapse presents a formidable challenge to experimental and computational studies. The latter requires two-phase flow solvers capable of capturing interactions between multiple deforming bubbles, pressure waves, formation of shocks, and their interactions with boundaries and turbulent vortical flows. Project researchers will use their award-winning, two-phase compressible flow code, CUBISM-MPCF, to capture the collapse of more than 50,000 bubbles interacting with a turbulent flow field at unprecedented resolution and performance.
During their first year, researchers used CUBISM-MPCF on Mira to successfully simulate a free-field collapsing cloud of 50,000 bubbles and a cloud of 20,000 bubbles in a homogenous turbulent flow. The 50,000-bubble simulation considered different geometrical arrangements (such as spherical or cylindrical), densities, and distributions (random or structured). These studies revealed that cavitation begins with the initiation of collapse at the bubble surface, followed by the formation of center-directed micro-jets that induce pressure up to 25 times higher than the ambient pressure.
Now, researchers are quantifying uncertainties in cloud peak pressures and collapse times under random initial conditions. Simulations of 5,000 to 10,000 bubbles permit 20,000 to 80,000 sources of randomness related to bubble radii, position, and vapor pressure. By exploiting the newly developed Multi-Level Monte Carlo method, a novel statistical sampling approach that is faster than its classical predecessor, researchers can simulate sources of randomness up to two orders of magnitude higher than similar state-of-the-art simulations.
Researchers predict that information gained from these simulations could revolutionize the development of engineering models for the prediction of the cavitation damage potential.