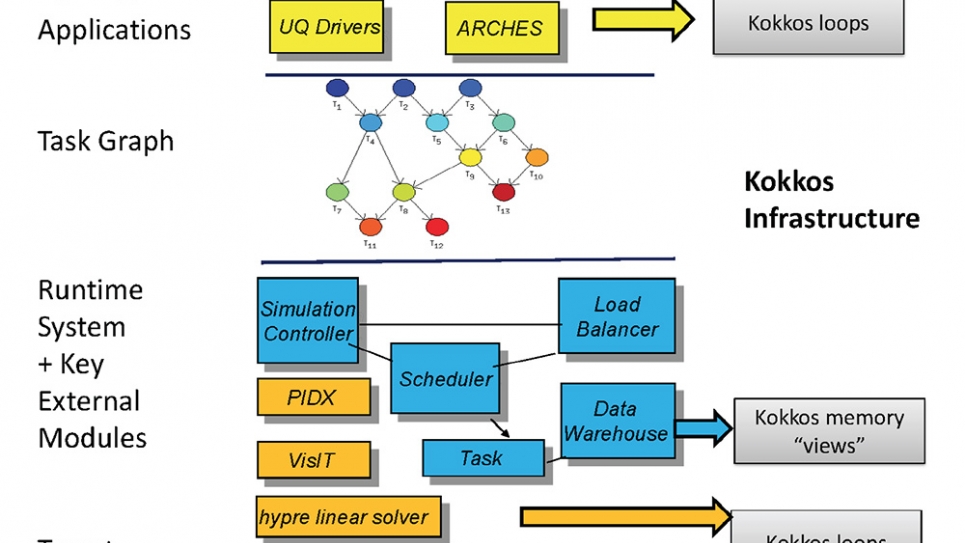
U.S. pulverized coal power plants with significant carbon greenhouse gas emissions currently account for 25 to 30 percent of the electrical power on the electrical grid, accounting for as much as 90 percent in some states. Modeling greenhouse gas formation and reduction from primary combustion modification is a multiscale and multi-physics problem with a strong dependency on a combination of many factors. Such challenges are opportunities for high-fidelity simulation techniques that can run on exascale resources while providing insight into the development of cleaner energy-production technologies. this project aims to use an industrially relevant, large-scale turbulent combustion application for realistic energy simulations, providing insight into the development of cleaner energy-production technologies.
U.S. pulverized coal power plants with significant carbon greenhouse gas emissions currently account for 25 to 30 percent of the electrical power on the electrical grid, accounting for as much as 90 percent in some states. Typical air emissions include particulate matter, sulfur oxides (SOx), nitrogen oxides (NOx), mercury (Hg), and carbon dioxide (CO2) as a greenhouse gas. U.S. regulations on several of these pollutants have led to primary combustion modifications and the addition of post-combustion flue-gas capture techniques. These methods can reduce the overall efficiency of the power cycle and drive up the cost of electricity, hence the need for reliable simulation predictions. For NOx reduction, the least disruptive retrofit option is primary combustion modification, which includes a variety of options like fuel staging and the use of over-fired air to modify the mixing characteristics and residence times in fuel rich and lean regions. These modifications affect the final outlet NOx.
Modeling NOx formation and reduction from primary combustion modification is a multiscale and multi-physics problem with a strong dependency on mixing rate, residence times, stoichiometric conditions, a wide range of chemical timescales, and varying fuel properties. Such challenges are opportunities for high-fidelity simulation techniques that can run on exascale resources while providing insight into the development of cleaner energy-production technologies.
To this end, this project aims to use an industrially relevant, large-scale turbulent combustion application for realistic energy simulations carried out at exascale. For this combustion application, the use of mesh scales that directly resolve more information on the mesh, such as the turbulent kinetic energy spectrum and reaction rate parameters for coal, will demonstrate higher-fidelity physics predictions than previously shown and start to make possible high-fidelity computational design in this technology, whose performance must be improved for numerous societal reasons. The simulation data and resulting simulation tool have the potential to impact boiler design by minimizing capital investment, increasing boiler efficiency by a 50 percent, and by association reducing CO2 greenhouse gases 50 percent.