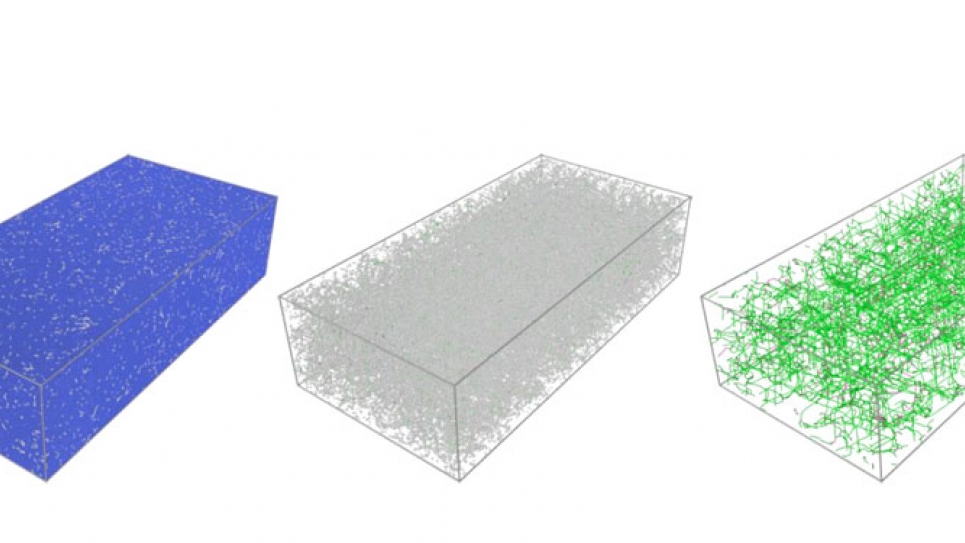
The research team will use large-scale molecular dynamics (MD) simulations to settle two long-standing controversies in classical physical metallurgy: (1) the microscopic origin of strain hardening, and (2) the nature and geometric character of dislocation patterns. Widely divergent theories have been advanced about these two phenomena, some “classical” theories even being mutually contradictory. The disarray persists because scientists are unable to test crystal plasticity properties while simultaneously (in situ) observing the underlying dynamics of atoms and dislocations, which are line defects in the crystal lattice known to be responsible for crystal plasticity.
In situ microscopy observations are possible only in thin electron-transparent films — where neither strain hardening nor dislocation patterns are observed. At present, MD simulations offer the only means to permit, in principle, simultaneous mechanical testing of bulk crystal plasticity in silico and fully detailed in situ observation of the underlying atomic dynamics. Because of their immense computational cost, direct MD simulations of crystal plasticity have been regarded as impossible. However, the team, in applying a newly established simulation capability, has demonstrated that direct cross-scale MD simulations of plasticity and strength of tantalum metal are feasible. The project objective now is to address three stages of strain hardening and the nature of dislocation patterns using cross-scale rather than multi-scale simulations. This effort will also increase understanding of the strength of structural materials.